While EVs are a definitive answer to cleaner mobility, environmental concerns related to battery production persists.
Published On Jun 12, 2023 08:00:00 AM
The EV invasion is in full swing, but does the impact of supplying huge amounts of cobalt, manganese and lithium – needed for batteries – threaten to make the mass switch an environmental and ethical own goal?
Composition of batteries
Lithium-ion batteries are highly complex things, explains David Greenwood, advanced propulsion systems expert at the Warwick Manufacturing Group, “An EV battery is a mix of materials. Some are conventional engineering materials, like steel and aluminium, and others are active materials: the electrochemical components.”
An anode (the negative electrode) is usually made from graphite with additives such as silicon. Graphite can be natural (mined and refined) or synthetic. The most expensive material in the battery is the cathode (the positive electrode), at about a third of the cost of the entire battery.
“The materials used vary: some are better for long-range vehicles, others are better for cheap, short-range vehicles and then there are some in between,” says Greenwood. “The dominant chemistries are nickel-cobalt-aluminium [NCA] and nickel-cobalt-manganese [NMC].
“Nickel isn’t a major supply problem: it’s already produced in huge quantities for the engineering industry and is used in stainless steel and many other things. Cobalt is the problem child. Most of it comes from the Democratic Republic of the Congo [DRC]. About 70 percent comes from large corporate mining companies that operate to worldwide standards, but about 30 percent are from small, informal mines which aren’t well regulated, and there are humanitarian concerns.”
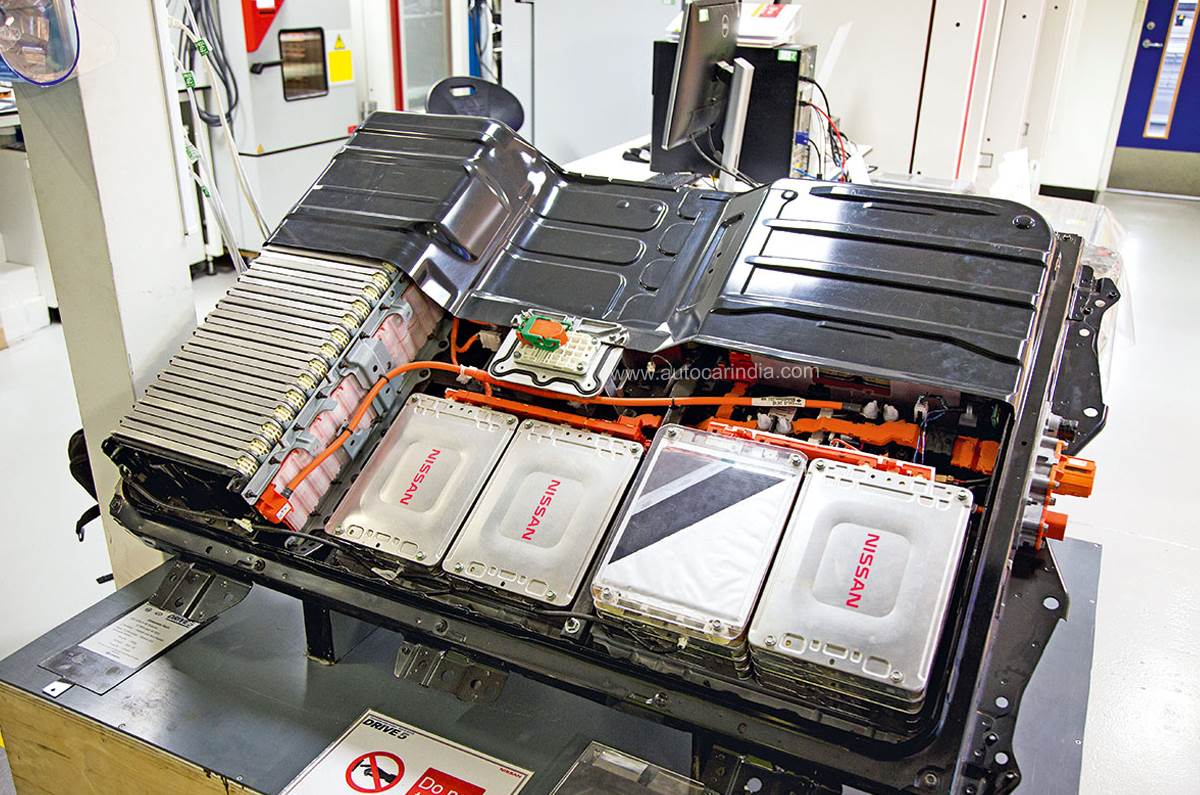
EV battery packs are complex to manufacture.
The amount of cobalt is being reduced, though. An NMC battery might once have contained six parts nickel, two parts cobalt and two parts manganese (a formula known as 622). Today, the state-of-the-art batteries are available with lesser cobalt and manganese compositions: nine parts nickel, a half part cobalt and a half part manganese (known as 811 or 955).
Batteries containing cobalt are typically used in long-range or high-performance vehicles and, until recently, in low-end and mid-range vehicles as well. Now a second category has emerged, the lithium-iron-phosphate (LFP) battery. It’s lower-capacity and less efficient than NMC, but contains no nickel or cobalt. ‘Doping’ it with manganese to produce an LMFP battery improves the performance.
In the future, there will be two battery categories. The first will be dominated by high-energy-density NMC batteries for long-range and high-performance vehicles and the second, cheaper LMFP batteries for low-cost, mid-range vehicles.
In the longer term, says Greenwood, “LFP may be replaced by the sodium-ion battery. At the high end and the least certain technology is solid state. It’s very promising, but not yet production-ready. We’re at least five or more likely eight years away from seeing solid-state batteries in the market.”
Mining of required minerals
Of the minerals on which existing technologies depend, the two most controversial are hugely abundant lithium and much rarer cobalt.
Lithium is mined in two ways, explains Caspar Rawles, data chief at Benchmark Mineral Intelligence: “The first is typical hard-rock mining. The ore is reduced to a 5.5-6 percent concentrate and then generally goes to China for chemical processing.” The ore yields the crystalline substance spodumene, which contains lithium.
The second is an extraction from high-altitude salt lakes called salars. “Lithium is leached from the surrounding geology over time and enriched in the ground,” explains Rawles. The lithium-rich brine collects naturally in underground lakes and then is pumped to the surface into evaporation basins on a huge scale. Once the brine is concentrated enough and over a period of a couple of years, it’s refined to extract the lithium.
The environmental impact of salars is controversial, and there’s concern that the brine extracted from underground is replaced by rainwater from the surface, causing droughts and affecting wildlife.
A new process in development could ease that situation in the future, but is some way off being commercially viable. “It’s called direct lithium extraction [DLE],” explains Rawles. “The lithium is extracted from the brine in real time, after which the brine is pumped back into the aquifers.”
EVs will massively increase the global demand for minerals like lithium and cobalt, says Rawles: “Supply chains are scaling up but nowhere near where they need to be for the energy transition.” The expectation is that supply chains will need to increase from a few hundred thousand tonnes to, in the case of some minerals, millions of tonnes by the end of this decade.
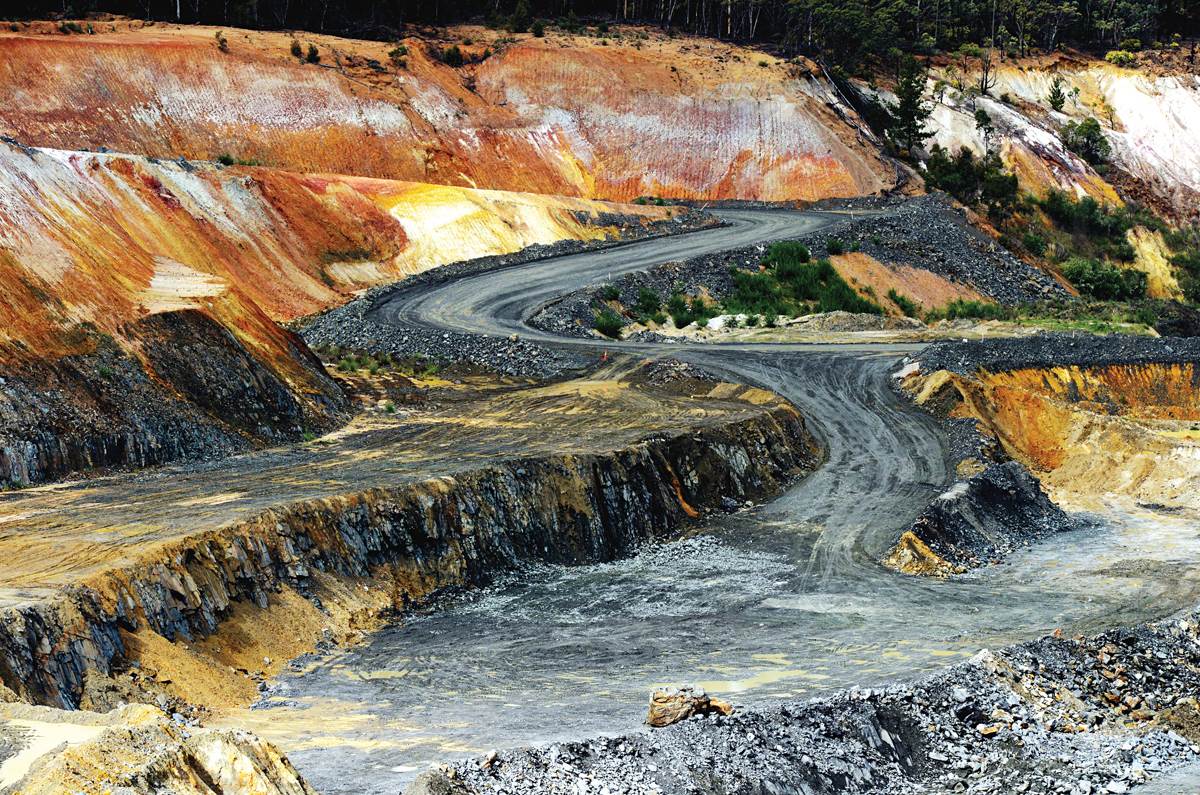
Australian open-pit mines supply much of the world’s lithium.
However, mining and mineral production aren’t new. Far from it, as Rawles points out: “There are risks associated with any kind of mining, whether it’s land contamination, emissions or correct rehabilitation of land. All these things are true for lithium, steel, aluminium, copper, cobalt… Anything mined out of the ground that goes into internal-combustion vehicles as well as EVs.”
More than half of the world’s lithium is mined in Australia, where mining is subject to strict environmental regulations.
Meanwhile, around 60 percent of the world’s cobalt comes from the DRC, where the mining of it has come under scrutiny. “The challenge in the DRC is that just over half of the production is from industrial mines producing massive volumes of copper and cobalt,” says Rawles. Then there’s a smaller group of industrial mines and thirdly the small artisanal mines (ASMs). “Some ASMs have been mining illegally, because they don’t have permission to extract the materials. There have also been instances of child labour,” he says. “In principle, there’s nothing wrong with small-scale mining by hand, and the livelihood of thousands of people depends on it. It just needs to be done safely and meet certain standards.”
The Congolese government has taken steps to regulate ASMs’ practices – for instance, mandating the 2019 creation of the Entreprise Générale du Cobalt by the country’s biggest mining firm, Gecamines.
Taking accountability
Car companies have taken their own steps to ensure traceability of the raw materials used in their batteries, too. As Greenwood points out, “The car industry is doing its utmost to manage supply chains, because the last thing it wants is another Dieselgate”.
Mercedes-Benz commissioned RCS Global to audit its supply chain for cobalt; BMW is on the board of the DRC-backed Responsible Cobalt Initiative; Volkswagen is working on a certification system for cobalt as part of the Responsible Minerals Initiative; Volvo in 2019 established a shared data network to ensure traceability of materials including cobalt; and this January, the Global Battery Alliance launched proof-of-concept pilots for a battery passport scheme to ensure that every battery made meets standards for human rights, child labour and emissions.
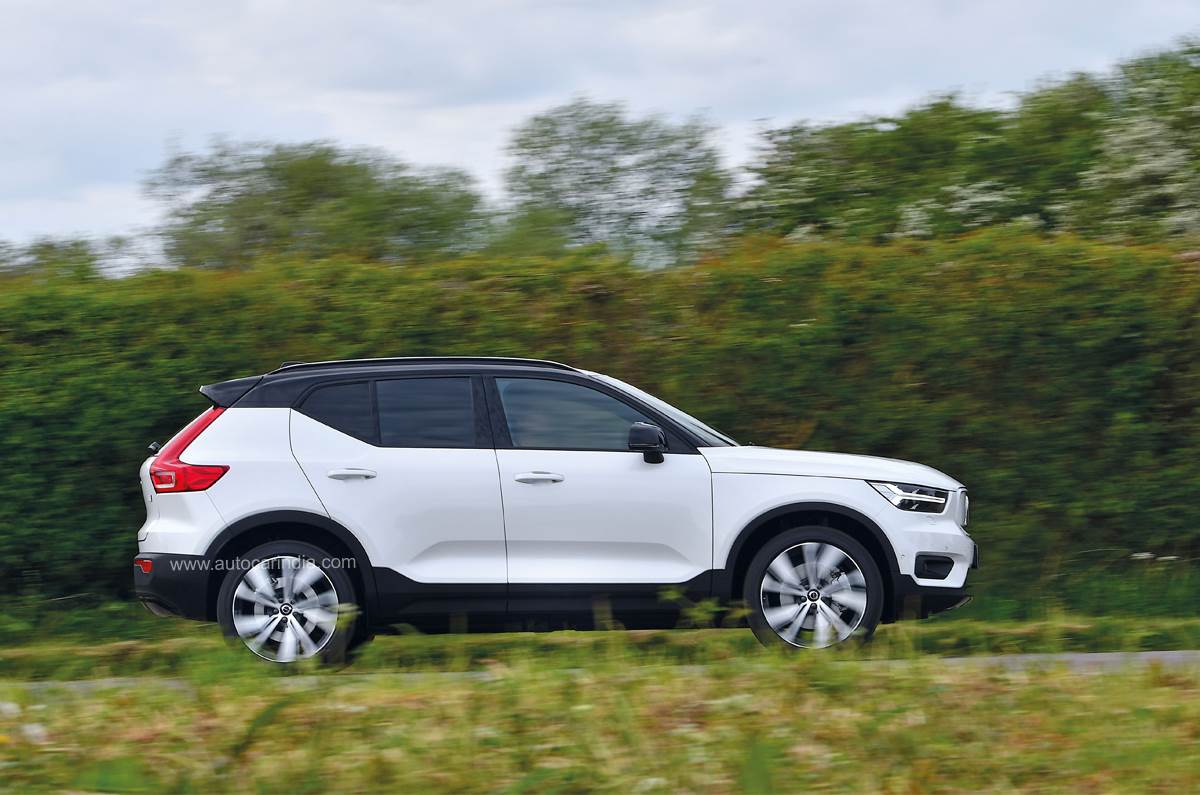
Volvo is one of many EV makers that trace their cobalt supplies.
Ultimately, these problems might be improved by new, lower-environmental-impact technologies that avoid lithium use entirely. One example is the sodium-ion battery that Greenwood mentioned earlier.
“Sodium is hugely abundant and can be taken from sea salt and lots of other sources,” he says. “It’s a low-cost, less mature technology than LFP, but it’s getting there. The energy density needs to improve, from around 160Wh/kg now to 180-200Wh/kg or so. It’s likely to appear in a stationary application first, then work its way into the car market from the bottom up.”
What seems clear is that the battery industry is evolving fast, both in manufacturing and performance of the technology.
“We aren’t using a science that has only just been discovered,” concludes Greenwood. “The best battery chemistry is only the best for four or five years, then something comes along and replaces it.”
Battery recycling
Lithium-ion battery recycling is already established, and all major carmakers are pursuing it, along with industrial firms and academic institutions like the Warwick Manufacturing Group.
In the EU, the Battery Directive already requires that at least 50 percent of batteries must be recycled at the end of their lives, but according to the WMG’s David Greenwood, that figure is being bettered, because tougher rules are on the way.
“Research is underway at [the] WMG on that, and we’ve filed some patents,” says Greenwood. “We hope to increase the recycling rate from 50 percent to 80-95 percent by the end of the decade.”
Future regulations are likely to dictate minimum recycled quantities of specific materials like lithium, as well as mandating the use of minimum quantities of recycled material in new batteries.
The recycling process involves a furnace extracting metals like cobalt in a process called pyrometallurgy. Water is used as a solvent for recycling the anodes, while the toxic solvent used for cathode materials, N-Methyl-2-Pyrrolidone (NMP), is trapped in a closed loop and reused.
The biggest problem isn’t the will to recycle, but a shortage of raw materials. Even first-generation Nissan Leaf batteries have proved so robust that most go to second-life stationary applications.
Also See:
Tech Talk: How much of an old car can you salvage for new ones?
Tech Talk: The brilliance of regen braking
Copyright (c) Autocar India. All rights reserved.